Soil sampling and analysis are vital for golf course superintendents to help determine effective and efficient turfgrass management programs. Soils are sampled to determine physical conditions, fertility (nutrient) status and chemical properties that affect their suitability as plant growing media.
Through a combination of field and laboratory research, analytical methods have been developed that provide quantitative estimates of plant-available nutrients. Field research determines the optimum soil test levels for various nutrients for specific soil and crop combinations. Optimum fertilizer practices can be determined by knowing the optimum test level of each nutrient for a specific crop and soil, and by knowing how much fertilizer is required to change soil-test values.
Soil testing is comprised of four steps:
- Collection of a representative soil sample;
- Laboratory analyses of the soil sample;
- Interpretation of analytical results; and
- Management recommendations based on interpreted analytical results.
Focusing on the first two steps, one should gain an understanding of the proper methods for collecting soil samples and of the potentials and limitations of soil testing.
Soil sampling
Soil testing begins with soil sampling. A soil analysis can be only as good as the sample sent to the laboratory. It’s important to realize only a tiny portion of a field is analyzed in the laboratory. For example, a one-pound soil sample collected from a five-acre field represents just 1/10,000,000 of the field. Therefore, it’s critical a soil sample be representative of the entire field.
SORTING THROUGH SOIL TESTING |
by Pat Jones The phrase comes out of the mouth of turf consultants, extension personnel, researchers and colleagues all the time: “The first thing to do is get a soil test.” Easy to say … not so easy to define. For the past few decades, golf course superintendents increasingly have understood that what’s underneath the playing surface dictates the health of the turf perhaps more than other factors. Soil tests, done properly, allow superintendents to balance nutrients, manage inputs and withstand stress better. The problem is that soil testing comes in many shapes and sizes and, more importantly, usually doesn’t provide a roadmap for fixing the problems they uncover. A soil test is like a medical diagnosis; it outlines the problem but doesn’t necessarily tell you what treatment is needed. That’s where superintendents’ knowledge or the use of soil consultants and other experts comes in to play. The cost of soil testing for golf courses can range from “free” (basic tests provided by chemical manufacturers or other vendors) to several thousand dollars a year for an ongoing program with an independent lab. Owners and clubs need to view soil testing as a necessary investment, not a luxury. To go back to the medical analogy, you can spend a lot of money treating the symptoms of the disease, or you can figure out the real problem and treat it. Soil testing sets a baseline for the entire agronomic program. Virtually every golf course superintendent has a file or a binder titled “soil tests” on his shelf. It’s no different than a corporation that spends a lot of time and money to create a strategic plan. Once all that effort is put into the process, the plan can sit on the shelf and gather dust or be a guide to implementing a program to succeed. Soils might be too acidic, too alkaline, nutrient-poor or nutrient rich. Or, there might be issues with the quality of the irrigation water on the site. The test is merely a first step in determining what fixes should be attempted to create a medium that supports turf grown at extremely low cutting heights. It could be necessary to add tons of solid minerals through topdressing or simply employ a different foliar nutrition program. It also might show that the program needs to incorporate more calcium, silica or magnesium in the mix. Every soil is different and every solution for the individual soil problem will be different. That’s the value of an ongoing testing program. Chances are good that two of the largest expenses in any golf course maintenance budget are fungicides and plant nutrition. Quite simply, healthy turf withstands stress better under warm, wet summer conditions and is less likely to need a heavy fungicide program. As a corollary, well-balanced soil provides many of the nutrients golf course turf needs. Instead of a shotgun approach to nutrition, superintendents can use a rifle. Start your soil testing program now and act on the results, and you and your turf will be less stressed out in the future. GCI |
The most common and economical method for sampling an area is composite sampling, where subsamples are collected from randomly selected locations in the field, and the subsamples are composited for analysis. The analytical results from composite sampling provide average values for the sampled area. The number of subsamples depends on field size and uniformity. Generally, a larger field or a less uniform field should be sampled more intensively than one that’s small and uniform. No less than five subsamples should be taken – 15 to 25 are preferred.
Alternatively, areas can be grid-sampled in a regular pattern. Each sample is analyzed separately so variability of soil properties can be determined. With data provided by grid sampling, maps of soil test values can be constructed from the results. This information can be entered into a geographical information system and combined with additional geospatial data, such as soil texture, crop yields and leaf analyses, and used in precision agriculture systems for variable application of fertilizers and other crop inputs. This is a much more expensive method of soil analysis because of the number of analyses required, although it provides valuable information about geospatial uniformity that can be used in precision agriculture.
Ideally, samples should be collected with a soil probe or auger, to the depth of tillage (usually 6 to 8 inches) or to the effective rooting depth of plants. Deeper samples might e collected for evaluation of subsoil properties, such as salt or nitrate accumulation. It’s helpful to sample to the same depth each time a soil is sampled so that year-to-year samples can be compared directly to monitor changes throughout time. A small shovel or trowel can be used if a probe isn’t available. Each subsample should be equal in size. The subsamples should be placed in a clean, plastic bucket and mixed thoroughly. Then the desired sample amount is removed from the bucket, and the remainder is discarded. Check with your testing laboratory to find out how large a sample it requires.
The area or size of the field sampled depends on management practices. Sample the smallest unit that will be managed separately. For example, if a field has two distinctly different halves, perhaps one half level and the other sloped, then sample the two areas separately, and fertilize each half separately to obtain optimum results. However, if each half of the area won’t be fertilized or managed individually, there’s no need for separate sampling. A single, representative sample will be less expensive and just as useful. Sample the smallest management unit.
Soil samples should be air-dried or taken to a test laboratory as soon as possible. To dry a soil sample, spread the soil out in a clean, warm, dry area, and let it dry for two to three days. It’s best not to heat or dry soil samples in an oven because soil chemical properties might be altered. Bag the sample and send it to a laboratory for analysis. Soil samples can be refrigerated for several days if they can’t be dried immediately.
Soil test values change slightly during the year, but the primary consideration for timing of soil sample collection is convenience. Collect samples early enough in the cropping cycle to allow results to be used to adjust management practices.
Soil-sample frequency depends on the specific soil test, environmental conditions and value of the crop. Status of some soil nutrients can change quickly, whereas others don’t. For example, phosphorus levels in soil are unlikely to change rapidly and annual testing might be unnecessary. Nitrogen levels, on the other hand, change quickly, and frequent tests are required to obtain accurate determinations of plant-available levels. A new soil analysis might be necessary after heavy rains or after a prolonged period of water-logging if one needs an accurate soil nitrogen level estimate.
When making substantial changes to soil fertility levels, it’s a good idea to make the change during a period of two to three years, retesting the soil annually. If a crop doesn’t have a high economic value, then occasional soil testing (once every three to four years) might be considered adequate in the absence of any noticeable nutritional problems. In contrast, commercial production of high-value crops might warrant annual testing to ensure maximum yields.
Soil analyses
After soil samples are received at a laboratory, a number of tests can be performed. A general understanding of soil testing will help you know how the results can be interpreted and appreciate the accuracy of the analytical results.
Soil supplies most of the mineral nutrition for higher plants through the plant’s root system. The root system extracts nutrients from the soil throughout a long period of time – two to three months for most annual crops, years for perennial crops. In contrast, a soil test determines the soil’s nutrient supplying capacity by mixing soil for only a few minutes with a strong extracting solution (often an acid or a combination of acids). The soil reacts with the extracting solution, releasing some of the nutrients. The solution is filtered and assayed for the concentration of each nutrient. The nutrient concentration then is related to field calibration research that indicates the yield level reached with varying soil nutrient concentrations. This method works well for some nutrients, but is less accurate for others, like those nutrients supplied largely from organic matter decomposition such as nitrogen and sulfur. This is primarily because of the difficulty of estimating or predicting the rate at which organic matter will decompose and release nutrients into plant-available forms.
Standard or routine soil tests vary from laboratory to laboratory, but generally include soil pH and available phosphorus (P) and potassium (K). They sometimes also include available calcium (Ca) and magnesium (Mg), salinity, and often include an analysis of organic matter content and soil texture. Most laboratories offer nitrogen (N), sulfur (S), and micronutrient analyses for additional cost.
The methods used to test soils vary depending on chemical properties of the soil. For example, tests used for measuring soil P are quite different in the acidic soils common in the southeastern U.S. than those used in the alkaline soils of the southwest. Analysis of southwestern soils with methods tailored for acidic soils will provide erroneous results. Therefore, it’s important to be aware of the methods used by test labs, and to select methods that are regionally appropriate. Local laboratories generally will use methods appropriate for your soils. A listing of local soil test laboratories might be found in the University of Arizona publication, “Laboratories Conducting Soil, Plant, Feed or Water Testing,” http://cals.arizona.edu/pubs/ garden/az1111.pdf .
Soil acidity
Soil pH is a measure of the acidity or alkalinity of a soil. The term pH technically only applies to solutions so the analysis must be conducted on a solution. Usually a soil sample is mixed with water, allowed to equilibrate for at least an hour, and the pH measured. Several factors affect pH measurement.
Primary among these is the salt concentration of a soil (a salt is any molecule that, when placed in water, separates into positively and negatively charged components or ions). The salt concentration of a soil might vary with the season or with fertilizer application. The salt concentration of a soil generally is greater immediately following fertilizer application than before. The result might be an apparent pH decline as much as one-half a pH unit.
When samples are collected frequently or at various times of the year, it might be noted pH values tend to increase and decrease, seemingly at random. This leads to questions regarding the reliability of soil pH measurements, but the fluctuations might be because of changes in soil salt levels. It doesn’t usually present a serious problem in the use of the analysis. Some laboratories measure pH in a dilute salt solution to mask salt-induced variations. This method gives lower pH values and the laboratory should provide interpretation guidelines.
Arizona soils generally are alkaline (high pH), and pH adjustment isn’t a common practice. In many other parts of the country, ground limestone is added routinely to soil to raise soil pH. In those parts of the country, lime requirement (amount of lime required to adjust the soil pH to a desired level) is determined. This test isn’t needed for alkaline Arizona soils.
Electrical conductivity
Electrical conductivity of a soil extract is used to estimate the level of soluble salts. The standard method is to saturate the soil sample with water, vacuum filter to separate water from soil and then measure electrical conductivity of the saturated paste extract. The result is referred to as ECe and is expressed in units of deciSiemens per meter (dS/m). Test laboratories might use differing soil-to-water ratios and use a multiplication factor to convert results to an ECe equivalent.
Electrical conductivity is a reliable test for soil salinity, and this is a routine test in the arid southwest. However, in wetter climates, electrical conductivity isn’t a standard test so, if soil samples are sent to a laboratory in another part of the country, electrical conductivity might have to be specifically requested.
Nitrogen
Nitrogen analyses aren’t difficult to conduct, but interpreting results can be problematic. This is because a considerable portion of soil nitrogen might be contained in the soil organic matter. Plant availability of organic nitrogen is dependent on organic matter breakdown, which is difficult to estimate. Therefore analyses of “total N”, a sum of all forms of soil nitrogen or organic nitrogen, aren’t routinely conducted. Instead, nitrogen in the nitrate form (NO3-N) is assayed. Nitrate is directly available to plants, so this test provides an indication of short term nitrogen availability. However, NO3-N can be lost quickly from soil, either leached past the rooting zone or lost to the atmosphere in gaseous forms.
The extractant used to remove NO3-N from the soil isn’t particularly important. Some laboratories extract NO3-N from soil with a salt solution, such as potassium chloride (KCl). However, other laboratories in the southwestern U.S. measure NO3-N in the same extract used to measure soil P (see below) to reduce analysis costs. Results from these two kinds of extractants are directly comparable.
Nitrate analyses can provide an accurate determination of the nitrogen available to plants at the time of soil sampling, although this might not provide reliable information concerning nitrogen availability later in the growing season. If soil nitrogen analysis is to be used for making fertilizer recommendations, it’s important the sampling be done shortly before planting time or during the growing season.
Phosphorus
Most soil phosphorus is tightly bound to soil particles or contained in relatively insoluble complexes. The phosphorus-containing complexes in alkaline soils are different than those in neutral or acidic soils. The amount of phosphorus removed during soil extraction is dependent on the nature of phosphorus complexes and on the specific extractant used, so it’s critical phosphorus extractants be matched to soil properties.
The Olsen or bicarbonate extractant, a dilute sodium bicarbonate solution, is used to extract phosphorus from calcareous, alkaline and neutral soils and is appropriate for Arizona soils. In contrast, most other phosphorus extractants, such as the Mehlich extractants, are suited for acidic soils and aren’t acceptable for arid-region soils. If an appropriate extractant is selected, phosphorus analysis is a reliable and useful soil test. On a soil test report, the analysis might be reported as PO4-P.
MAJOR EXCHANGEABLE CATIONS
The four major exchangeable cations in arid-region soils are potassium, calcium, magnesium, and sodium. All except sodium are essential plant nutrients; however, sodium is included because it plays an important role in soil physical properties. Soil sodium level also is needed for calculations of cation exchange capacity and exchangeable sodium percentage.
An ammonium acetate extractant is used to extract only exchangeable potassium, calcium, magnesium, and sodium from arid-region soils but not less plant-available forms. Some difficulty might be encountered in soils containing calcium or magnesium carbonates (calcareous soils) because the ammonium acetate extraction might remove some calcium or magnesium from these minerals along with the exchangeable forms.
In these situations, the analytical results might indicate slightly elevated levels of these nutrients. This isn’t usually a large problem and potassium, calcium and magnesium tests generally provide excellent estimates of plant available levels of these nutrients.
Cation exchange capacity
Cation exchange capacity might be estimated as the sum of the major exchangeable cations (potassium, calcium, magnesium, and sodium). Most laboratories don’t routinely conduct a separate analysis for cation exchange capacity but use the ammonium acetate extractable levels of these elements (discussed above) for this calculation.
ESP and sAR
Exchangeable sodium percentage and sodium adsorption ratio are measures of soil sodium content relative to other soil cations. Exchangeable sodium percentage is the concentration of sodium divided by the cation exchange capacity. As described above, the cation exchange capacity often is estimated as the sum of the major exchangeable cations, so exchangeable sodium percentage equals Na/(K+Ca+Mg +Na). Sodium adsorption ratio is roughly comparable to exchangeable sodium percentage but is a ratio of sodium to calcium plus magnesium. For this calculation, concentrations of sodium, calcium and magnesium are measured in a saturated paste extract (see discussion of electrical conductivity above). The equation used for calculation of sodium adsorption ratio is: Na+ divided by the square root of Ca2+ plus Mg 2+ where concentrations are in units of mmol/L. Sodium adsorption ratio and exchangeable sodium percentage are useful measures of the influence of sodium on soil properties. The choice between the two is based largely on the type of extraction used for cation analyses. Sodium adsorption ratio can be used with either soil or water samples, whereas exchangeable sodium percentage is applicable only with soils.
Free lime
Free lime is a measure of soil carbonates (salts of CO32-). When combined with an acid, carbonates release gaseous carbon dioxide. The test usually performed for soil carbonates is semiquantitative. A weak acid solution is applied to the soil sample, and the degree of fizzing or release of carbon dioxide gas is determined visually and categorized as none, low, medium or high.
Sulfur
Sulfur, like nitrogen, might be contained primarily in the soil organic matter, but plants absorb only the inorganic sulfate (SO42-) form. Measuring total soil sulfur doesn’t provide a good estimate of sulfur plant availability because rates of release from organic matter can’t be accurately predicted.
Instead, sulfur in the sulfate form is a more common measure. Sulfate can be extracted from the soil with several extractants, including water or weak salt solutions. Analysis of SO42- is relatively easy, but it provides a measure of immediately available sulfur, and not the soil’s long-term ability to supply sulfur to a growing plant.
Micronutrients
Micronutrient analysis is optional at most laboratories. Most laboratories in the southwest region use a DTPA-TEA (diethylenetriamine pentaacetic acid - triethanolamine) extractant, which uses the chelating agent DTPA that’s tailored to extract available iron (Fe), copper (Cu), manganese (Mn), and zinc (Zn) from calcareous soils.
Analyses of these micronutrients probably are less accurate for predicting the likelihood of plant deficiencies or of crop responses to supplemental application of these nutrients than analyses of macronutrients such as potassium, calcium and magnesium because of the influence of dynamic soil conditions. For example, manganese availability can change substantially if the soil drainage status is altered, becoming more available in waterlogged soils and less available in dry soils. Iron availability also is affected by soil moisture and irrigation practices. Furthermore, availability of copper, iron, manganese, and zinc are greatly affected by soil pH, so soils might need to be retested if soil pH is significantly altered. Soil testing can’t predict the effects of altering management practices on availability of these nutrients reliably.
The most common method of extracting boron (B) from soils is with hot water. The plant availability and level of extractable boron in a soil might be influenced by soil pH. Boron also might be leached from the rooting zone. Therefore, extractable soil boron provides estimates of plant availability that are less reliable than those of many other nutrients.
Few laboratories conduct soil molybdenum (Mo) analysis. Molybdenum is present at low levels in most soils, much lower than most of the other nutrients, making an accurate determination difficult. Most plants have a low requirement for molybdenum, and slight differences in soil molybdenum levels can impact plant performance. Also, seeds might contain enough molybdenum to supply a crop’s entire molybdenum requirement. For these reasons soil tests for molybdenum are of limited use and are seldom conducted.
Organic matter
The organic matter level of a soil can be determined by several analytical techniques, all of which are accurate. All measure the amount of soil organic matter, but most don’t determine its nature or how it will contribute to soil fertility. Levels of nutrients contained in the soil organic matter can be determined (but usually aren’t), and rates of mineralization or nutrient release from organic matter can’t be predicted reliably because of the influences of weather and climate. Organic matter content isn’t routinely determined in southwestern soils because the levels are relatively low and normally change only slightly.
Maximizing nutrient use
Soil analyses can provide information that’s important for maximizing nutrient use efficiency and agricultural productivity. Furthermore, a historical record of soil properties provided by long-term soil testing is useful for determining the effectiveness of fertilizer management strategies.
Soil sampling is the critical first step in a soil testing program. The second is selection of a laboratory that will use analysis procedures appropriate for regional soils and conditions. Third, an understanding of the accuracy and limitations of individual procedures and of the meaning of soil test results is essential. The last steps, interpreting soil analysis values and developing a fertilizer management program, are crop specific and sometimes dependent on additional soil and climatic properties. GCI
James L. Walworth is associate professor and soil specialist at the University of Arizona, College of Agriculture and Life Sciences in Tucson, Ariz.
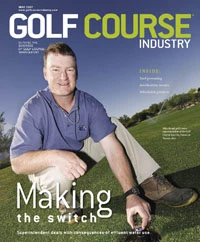
Explore the May 2007 Issue
Check out more from this issue and find your next story to read.
Latest from Golf Course Industry
- GCSAA names Grassroots Ambassador Leadership Award winners
- Turf & Soil Diagnostics promotes Duane Otto to president
- Reel Turf Techs: Ben Herberger
- Brian Costello elected ASGCA president
- The Aquatrols Company story
- Albaugh receives registration for chlorantraniliprole
- Honored by the association he helped expand
- The Carolinas GCSA Conference and Show: 5 W’s preview