In many southern regions of the United States, the most frequently encountered turfgrass is common bermudagrass (Cynodon dactylon) or one of the many interspecific hybrid cultivars, which are crosses of common and African bermudagrass (Cynodon transvaalensis). Bermudagrass is a warm-season turfgrass that thrives in the south during warm months when cool-season grasses are often heat stressed. In much of the turfgrass transition zone, where both cool and warm-season grasses can grow, bermudagrass will enter dormancy during cold months and appear dead or brown. As soil temperatures warm in the spring, the grass will resume growth and the stand will transition from brown to green. There are numerous traits of bermudagrass that make it an ideal turf species for the southern regions. The turf can tolerate heat, periods of drought, elevated salts, and it has a spreading growth habit that makes it well adapted to for many different applications such as lawns, athletic fields, fairways and greens. In addition, compared to other grasses, bermudagrass has fewer insect pests or diseases that can degrade the quality and appearance of a lawn or fairway.
Of the few bermudagrass diseases, spring dead spot (Figure 1) is the most devastating and difficult to manage in the southern United States where the grass goes dormant during cool winter months (Smiley et al). The disease is caused by three similar fungi all in the genus Ophiosphaerella (pronounced ‘O-fee-o-sfair-rella’). Geographic location strongly influences which species can be found causing the disease in various regions of the United States. For example, in central lower Midwestern states Ophiosphaerella herpotricha is often the most frequent species encountered; in California, Atlantic and Eastern Gulf Coast states Ophiosphaerella korrae is the most common species associated with bermudagrass. Ophiosphaerella narmari is much less common than the other two species and occasionally has been found in regions such as California or Oklahoma. However, the fungus cannot be identified to species accurately based on disease symptoms and must be isolated into culture in a laboratory.
Spring dead spot causes the development of unsightly dead, sunken patches in bermudagrass stands in the spring as the grass resumes growth. The dead patches can persist for many weeks to months and recovery requires healthy grass to regrow into the damaged areas. The dead patches also can permit the establishment of weeds. In addition to the cost of weed removal from the patches, cultural management efforts also are required to encourage reestablishment of the grass into the affected areas. Not all stands of bermudagrass are equally affected by the disease. Bermudagrass cultivars can vary in the severity of disease with the more cold tolerant cultivars having less damaged than those that are not cold tolerant (Baird). Management also can influence disease incidence and severity. Lawns that are infrequently fertilized, irrigated or treated with herbicides may not develop symptoms of disease, but if present, symptoms are less severe and not readily apparent due to the presence of weeds.
Three things must be present for a turfgrass disease to occur: a susceptible host plant, a virulent pathogen (a fungus in this case) and environmental conditions conducive for disease development. Together these are known as the disease triangle. Change or remove one side of the triangle and the disease will not occur. For spring dead spot, soil temperature is the primary environmental factor that influences the host-fungus interaction and the development of disease. Cool soil temperatures allow the fungus to somehow reduce the ability of the plants to survive cool temperatures and/or cold temperature-induced dormancy. Since it’s not feasible to alter soil temperature, we must look at ways to influence the plant side of the disease triangle to more effectively manage this disease. We know that bermudagrasses can vary in susceptibility to spring dead spot and reduced disease has been associated with the cold tolerance of some bermudagrass varieties. Unfortunately, almost no information is available about how these factors affect the interaction of the pathogen with its host. This basic information is crucial for the efficient design of more effective disease control strategies. Therefore, we set out to investigate how the differences among bermudagrasses influence disease. What follows is a description of our findings on the interactions of the spring dead spot fungus, O. herpotricha, with tissues of bermudagrasses differing in susceptibility to this disease.
Findings
A limitation to studying turfgrass root diseases is the inability to easily study the plant-fungus disease interaction because it happens below ground and often inside of roots. To study the interaction, an approach to grow bermudagrass from stolon segments without soil was developed (Figure 2). Then genes, pieces of DNA that code for traits, were inserted into the genetic material of the fungus, O. herpotricha. We chose to use two fluorescent reporter genes (red and green) to visually label the fungus, allowing us to see the otherwise difficult-to-view interaction. The gene responsible for green fluorescence was originally isolated from a jellyfish and the gene for the red fluorescence from a marine coral. The technique of using green or red fluorescence has been used by researchers worldwide to observe microbes. These genetically modified, fluorescent fungi were then inoculated onto three cultivars of bermudagrass: Tifway 419, a susceptible interspecific hybrid; Midlawn, a more resistant hybrid; and Uganda, an African-type bermudagrass with an unknown response to spring dead spot that was anticipated to be resistant (Martin). We examined how the fungus entered, colonized and caused the necrosis of the root and stolon tissues of the three bermudagrasses under cool temperature conditions.
We observed differences between the cultivars that have never been reported. For example, the root surface epidermal and internal cortical cells of Tifway 419 and Midlawn were rapidly colonized by the fungus (Figure 3); however, the internal central vascular region remained uninfected. In contrast, both the roots’ surface and all internal tissues (cortex and vasculature) of Uganda were colonized. In general, Tifway 419 roots exhibited greater colonization and necrosis than the more tolerant cultivar Midlawn. On the other hand, though its roots were heavily colonized, Uganda exhibited very little necrosis. Based on these results we were able to observe that the fungus colonized the plants differently and plants varied in their responses to the fungus. These responses correspond to the severity of disease symptoms seen in field plantings of Tifway 419 and Midlawn.
Next, the stolons of these three bermudagrasses were inoculated with the fluorescent fungi, but resulted only in very limited epidermal and cortical colonization and necrosis by fungus (Figure 4). However, if stolons had been wounded, the fungus invaded such openings resulting in internal necrosis of Tifway 419 stolons and a slightly less severe internal necrosis of Midlawn stolons. However, even though wounded Uganda stolons were extensively colonized by the fluorescent fungi, no necrosis was apparent (Figure 5). These results suggest the fungus can persist a long time associated with the surface of stolons without causing much disease. It’s also important to note that cultural management activities that damage stolons, such as verticutting, during cool periods that favor fungal growth, could increase the disease’s incidence or severity.
We also have used confocal scanning laser microscopy, which uses lasers to peer inside the infected plant and generate "optical sections" of the tissues, similar in concept to MRI or CAT scans. A computer converts the information from the lasers into scan-like images of the fungus inside the bermudagrass (Figure 6). The resulting scans can be examined one at a time or stacked to produce 3-D images of the fungus on the surface of or within the plant. Using this technique, the fungus appears to be able to grow deep inside the plant, in spaces between cells (Figure 7), before invasively colonizing the adjacent host cells. This suggests the fungus can obtain nutrients from its hosts’ cells without immediately damaging these cells.
These studies have allowed us to propose various explanations and ask new questions concerning some of the observations made about the disease. For example, why does greater cold tolerance appear to reduce the severity of disease? It’s possible breeders are introducing more traits than just cold tolerance from African bermudagrasses, like Uganda. In hybrids with common bermudagrasses, these additional traits reduce the plant’s deleterious necrotic response to the fungus, making it more resistant. Is the necrotic response of susceptible cultivars, such as Tifway 419, an effort by the plant to stop the fungus? As a result, does the plant unintentionally doom itself by allowing the necrotizing fungus to gain even greater access to its food or plant starch reserves (Figure 7)? Does the fungus "hibernate" in the spaces between plant cells during summer months when soil temperatures are not conducive for growth? Current efforts are attempting to answer these questions and further our understanding of the plant-fungus interaction.
In addition to advances in understanding the disease, the previously described techniques could be used to evaluate new experimental cultivars for resistance to spring dead spot. Traditionally this evaluation process is a very slow one requiring many years to complete. For the traditional method, 2- to 3-year-old stands of bermudagrass are inoculated with the fungus. Symptom development requires one to two years before they are severe enough to accurately rate. Once symptoms are present, in the now 3- to 5-year-old stand, disease severity can be evaluated over the next two to three years. As bermudagrass breeding efforts continue at Oklahoma State University, we hope to use the genetically modified, fluorescent fungi in a streamlined laboratory assay to rapidly screen new experimental cultivars for resistance (or tolerance) to spring dead spot. This would greatly improve our ability to enhance and deploy resistance as the predominant control strategy for this disease.
Summary
Spring dead spot of bermudagrass is a challenging disease to manage and understand. Here we’ve used state-of-the-art molecular approaches to genetically engineer one of the fungi that causes the disease so we can better observe and understand the fungus-plant interaction. We’ve observed that the fungus interacts differently with different bermudagrasses and that the host plant’s response to infection could serve as its Achilles heel. Future efforts will continue to investigate how the fungus colonizes the plant and how the plant responds to the fungus. This work will make possible the breeding of elite bermudagrass cultivars with durable resistance to this important disease. This would greatly reduce the added costs of cultural and chemical management of the disease. Successful use of the fluorescent fungi in this study is very encouraging and suggests that the same strategy can be applied to the other species of Ophiosphaerella causing spring dead spot. GCI
Oliver Caasi, M.S., was a research associate, and Nathan R. Walker. Ph.D., and Stephen M. Marek, Ph.D., are associate professors in the Department of Entomology and Plant Pathology at Oklahoma State University in Stillwater, Okla. Thomas K. Mitchell, Ph.D., is an assistant professor in the Department of Plant Pathology at Ohio State University in Columbus, Ohio.
Literature Cited
Baird, J. H., Martin, D. L., Taliaferro, C. M., Payton, M. E., and Tisserat, N. A. 1998. Bermudagrass resistance to spring dead spot caused by Ophiosphaerella herpotricha. Plant Dis. 82:771-774.
Martin, D. L., Tisserat, N. A. Bell, G. E., Taliaferro, C. M., Baird, J. H., Kuzmic, R. M., and Dobson, D. D. 2000. Spring dead spot resistance of African bermudagrass selections. P. 150 In 2000 Agron. Abs. ASA, Madison, Wis.
Smiley, R. W., Dernoeden, P. H., and Clarke, B. B. 2005. Compendium of turfgrasses diseases, 3rd ed. The American Phytopathological Society. St. Paul, Minn.
Acknowledgement: The authors thank the United States Golf Association for their support of this project.
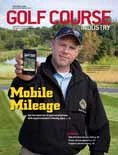
Explore the October 2009 Issue
Check out more from this issue and find your next story to read.
Latest from Golf Course Industry
- Editor’s notebook: Green Start Academy 2024
- USGA focuses on inclusion, sustainability in 2024
- Greens with Envy 65: Carolina on our mind
- Five Iron Golf expands into Minnesota
- Global sports group 54 invests in Turfgrass
- Hawaii's Mauna Kea Golf Course announces reopening
- Georgia GCSA honors superintendent of the year
- Reel Turf Techs: Alex Tessman