The goal of every turfgrass manager is to provide a playable surface and aesthetically pleasing green turfgrass. Achieving the latter involves a reciprocal balance between soil, fertility, moisture, temperature, humidity, grass species, mowing techniques, cultural practices and cooperation from Mother Nature. All these aspects have to be working in sync for turfgrass to perform properly and be appealing colorwise.
Protecting and strengthening chloroplasts would seem like the logical action to take because this is where chlorophyll, a pigment that gives turfgrass its green appearance, is developed.
The most important characteristic of plants is their ability to photosynthesis – to make their own food by connecting light energy into chemical energy. This process is carried out in specialized organelles called chloroplasts. A photosynthetic cell contains anywhere from one to several thousand chloroplasts. The electrons from chlorophyll molecules in photosystem II replace the electrons that leave chlorophyll molecules in photosystem I.
Located inside the chloroplast are thylakoid membranes where light reactions take place. This is where chlorophyll is found, therefore, there’s a synergistic relationship between keeping the chloroplasts and the thylakoid membranes as healthy as possible.
There are events that can be harmful to chloroplasts and thylakoid membranes, as well as necessary components that can prevent damage to them.
Free radicals
One event that can damage chloroplasts is the development of free radicals. The medical profession has shown that free radicals can cause diseases in the human body. Likewise, turfgrass managers know that research throughout the past several years has shown free radicals can damage lipids, proteins and DNA inside cells of turfgrass plants, including chloroplasts.
Typically, free radicals are stable molecules that contain pairs of electrons. When a chemical reaction breaks the bonds that hold the paired electrons together, free radicals are produced. They contain an odd number of electrons, which make them unstable, short-lived and highly reactive. As they combine with other atoms that contain unpaired electrons, new radicals are created, and a chain reaction begins (Droge, 2002; Haag, 2005).
This chain reaction, or accumulation of reactive oxygen species, in plants is generally ascribed to several possible sources (Klessig and Malamy, 1994; Corpas et al.,2001; Desikan et al., 2001; Blokuna et al., 2003): cell-wall-bound perxidases, membrane-located NADPH oxidases, amine oxidases, xanthine oxidase, chloroplastic electron transport chains, mitochondrial electron transport chains, and peroxisomal fatty acid B-oxidation, which includes the H202-generating argyl-coenzyme A oxidase steps (Couee et al., 2006). These sources can be attributed to environmental causes such as drought, heat, and ultraviolet light, or chemicals such as herbicides (Haag, 2005).
Accumulation of reactive oxygen species is central to plant response to several pathogens. One of the sources of reactive oxygen species is the chloroplast because of the photoactive nature of the chlorophylls (Kariola et al.,2005). The free radicals, or reactive oxygen species, are singlet, hydroxyl, superoxide and hydrogen peroxide.
Light
There’s a catch-22 with light. Light is necessary for photosynthesis to occur; however, it also can play a part in the degradation of chlorophyll.
When photosynthetic organisms are exposed to ultraviolet radiation, significant, irreversible damage to important metabolic processes within the cell might occur (such as lesions in DNA and inhibition of photosynthesis). Through these reactions and others, radical forms of oxygen are often created. Many reports suggest this damage is because of oxidative stress resulting from UV-A, (Dring et al., 1996, Jeffrey and Mitchell, 1997, Turcsanyi and Vass, 2000) UV-B (Teramura and Ziska, 1996, Gotz et al., 1999, Mazza et al., 1999, hideg et al., 2000, Estevez et al., 2001) or both (Krause et al., 1999, Muela et al., 2000, Vega and Pizzaro, 2000, Laloi et al., 2006).
Photosynthetic light absorption and energy usage must be kept in balance to prevent formation of reactive oxygen species in the chloroplasts. Drought causes stomatal closure, which limits the diffusion of carbon dioxide to chloroplasts and thereby causes a decrease of carbon dioxide assimilation in favor of photorespiration that produces large amounts of hydrogen peroxide (Noctor et al., 2002). Under these conditions, the probability of singlet oxygen production at phtosystem II and superoxide production of photosystem I is increased (Niyogi, 1999; Foyer et al., 2005). These can cause direct damage or induce a cell suicide program (Tambussi et al., 2000).
It has been known for a long time wavelengths in the ultraviolet-B region of the spectrum are effective in inactivating photosynthesis, and the molecular target is photosystem II (Jones and Kok, 1966., Chen and Gallie, 2005). An excess of light brings about the inactivation of oxygenic photosynthesis, a phenomenon known as photoinhibition (Powles, 1984), and the molecular target of photoinhibition is photosystem II, a thylakoid multisubunit pigment-protein complex (Bergo et al., 2003). The major effect of ultraviolet-B light on the thylakoid proteins is the breakdown of the reaction centre D1 protein (Trebst and Depka, 1990; Friso et al., 1994; Barbato et al., 1995).
One must question whether ultraviolet-B radiation will become an even more serious factor in the future. The depletion of the stratospheric ozone is causing renewed concern about the increased level of ultraviolet-B radiation reaching the earth’s surface (Smith et al., 1995). It’s also known exposure to environmental ozone can cause significant damage to turfgrass by imposing conditions of oxidative stress (Chen and Gallie, 2005; Grimes et al., 1983; Schraudner et al., 1998). This might be the case because we’re seeing a gradual increase in yearly temperatures throughout the world and an increase in skin cancers in humans. How it affects crops and turfgrass plants in the future remains to be seen.
Senescence
Senescence results in massive levels of cell death, but the purpose of senescence isn’t cell death; rather death only occurs when senescence has been completed. Senescence occurs in two stages. The first stage is reversible, and the cells remain viable throughout. The second stage results in cell death (Buchanan-Wollston et al., 2003; McGlaughlin and Smith, 1995; Mothes et al., 1960; Riefler et al., 2006; Venkatrayappa et al., 1984).
The key enzyme in the pathway to chlorophyll degradation during senescence appears to be pheophorbide a oxygenase. The activity of pheophorbide a oxygenase increases dramatically during senescence, implicating this enzyme as a control point in the process (Buchanan-Wollston et al., 2003; Hortensteiner et al., 1998). Light absorption by pheophorbide a oxygenase also is believed to cause the production of singlet oxygen (Pruzinska et al., 2005), which is a free radical.
Because senescence is reversible, it suggests that fully developed chloroplasts retain enough genetic information to support regreening and chloroplast reassembly.
Calcium and potassium
From a nutritional standpoint, there are various nutrients and compounds that can be applied in the process of strengthening and defending chloroplast damage.
Because the chloroplasts and thylakoid membrane are located inside the plant cell, the first line of defense would seem to be to strengthen the plant cell by keeping calcium and potassium at optimal levels. Calcium plays a key role in strengthening the cell walls of the turfgrass plant, while potassium helps strengthen cell walls inside the turfgrass plant, which makes it harder for physiological problems to occur inside the cell wall (Haag and Serrato, 2006).
With regard to calcium applications, add a light amount of zinc along with the calcium because zinc helps calcium to translocate to the cell walls (Haag and Serrato, 2006).
Amino acids
Amino acids are the building blocks of proteins. Under optimal conditions, proteins are able to perform the normal physiological function to synthesize amino acids, but intensively manicured turfgrass, such as golf courses and athletic fields, are rarely operating under optimal conditions because of stress caused by low mowing heights and traffic (Haag and Serrato, 2006).
To date, 154 proteins in the turfgrass plant have been identified – 76 (49 percent) are integral membrane proteins. Twenty-seven new proteins without known functions, but with predicted chloroplast transit peptides, have been identified – 17 (63 percent) are integral membrane proteins. These new proteins are likely to play an important part in thylakoid biogenesis (Friso et al., 2004).
The application of amino acids plays an extremely important part in developing the proteins specifically designed to help chloroplasts, thylakoid membranes, photosystem I and photosystem II to function properly. These proteins are known as D1, D2 CP43, CP47 (de Weerd et al., 2002, Zheleva et al., 1998) and cytochrome b559. Of special importance is the D1 protein because it exhibits the highest turnover rate of all the thylakoid proteins (Matto, 1984; VonWettstein et al., 1995; Prasis et al., 1992; Aro et al., 1993; Schuster et al., 1988) and is highly vulnerable to singlet oxygen (Barber, 1984), a free radical.
Antioxidants
The antioxidants a-tocopherol (vitamin E), ascorbic acid (vitamin C), carotenoids (B-carotene), vitamin B6 and mannitol in some biostimulants play a vital role in scavenging free radicals (Barna et al., 2003) and helping protect chloroplasts, thylakoid membranes inside the chloroplasts, photosystem I and photosystem II.
The best biostimulant that I’ve encountered to date is the N.O.G. product.
Carotenoids (B-carotene)
In terms of its antioxidant properties, carotenoids can protect photosystem I and photosystem II in one of four ways: (i) by reacting with lipid peroxidation products to terminate chain reactions (Burton and Ingold, 1984; DellaPenna and Pogson, 2006); (ii) by scavenging singlet oxygen and dissipating the energy as heat; (iii) by reacting with triplet or excited chlorophyll molecules to prevent formation of singlet oxygen, or (iv) by dissipation of excess excitation energy through the xanthophyll cycle (Mathis and Kleo, 1973).
Xanthophylls function as accessory pigments for harvesting light at wavelengths that chlorophyll can’t and transfer the light energy to chlorophyll. But, they also absorb excess light energy and dissipate it to avoid damage in the xanthophyll cycle.
a-tocopherol (vitamin E)
A-tocopherol (vitamin E) is considered a major antioxidant in chloroplasts in at least two different but related roles. It protects photosystem II from photoinhibition and thylakoid membranes from photooxidative damage (Havaux et al., 2002; Havaux et al., 2005; Delong and Steffen, 2002, Flohe and Traber, 1999). The antioxidant properties of vitamin E are the result of its ability to quench singlet oxygen and peroxides (Fryer, 1992; Sattler et al., 2006).
Although vitamin E is a less efficient scavenger of singlet oxygen than B-carotene, it might function in the thylakoid membrane to break carbon radical chain reactions by trapping peroxyl radicals (Fryer, 1992; Burton and Ingold, 1984; Mathis and Kleo, 1973).
Ascorbic acid (vitamin C)
It’s generally believed maintaining a high ratio of ascorbic acid is essential for the scavenging of free radicals (Mitler, 2002) and are needed in high concentrations in the chloroplasts to be effective in defending the turfgrass against oxidative stress (Noctor and Foyer, 1998). Although ascorbic acid can directly scavenge the free radicals superoxide and singlet oxygen, the main benefit ascorbic acid plays in the prevention of free radicals is that it’s an excellent scavenger of the hydroxyl radical (Blokhina et al., 2002; Yoshida et al., 2006). The hydroxyl radical is dangerous to turfgrass because it can inhibit carbon dioxide assimilation by inhibiting several Calvin cycle enzymes (Asada, 1996).
Vitamin B6
Apart from its function as a cofactor, vitamin B6 is also thought to act as a protective agent against reactive oxygen species, such as singlet oxygen (Bilski et al.,2000; Chen and Xiong, 2005; Ehrenshaft et al.,1999; Drewke and Leistner, 2001). Vitamin B6 is also the master vitamin in processing amino acids and plays an important role in developing proteins specifically designed to help chloroplasts, thylakoid membranes, photosystem I, and photosystem II to function properly.
Mannitol
The antioxidant mannitol has the ability to protect and quench two damaging free radicals: singlet oxygen and hydroxyl. Singlet oxygen is damaging because it can react with proteins, pigments and lipids and is thought to be the most important species for light-induced loss of photosystem II activity, as well as the degradation of the D1 protein (Krieger-Liszkay, 2004). It has been demonstrated that when mannitol is present in the chloroplasts, it can protect plants against oxidative damage by the hydroxyl radicals (Senn, 1987; Shen, 1997).
Manganese and magnesium
Both of these nutrients are attached to the chlorophyll molecule that’s located inside the chloroplasts. These two nutrients play a part in making turfgrass greener by helping develop chlorophyll. They also transport other vital nutrients and are responsible for many enzymatic functions and help prevent chlorophyll degradation in the cells (Haag and Serrato, 2006).
Carbon
There’s new evidence carbon plays a role in the development of the turfgrass plant leaf, and that a reduction in carbon reduces photosynthetic activity, which reduces carbohydrate availability to the turfgrass plant. There’s also new evidence to suggest proper development of the turfgrass plant can’t occur without proper amounts of carbon in the chloroplast (Raines and Paul, 2006). There’s more evidence to suggest that, if there’s an abundant source of carbon in the thylakoid membranes inside the chloroplasts, it can be mobilized for use as an energy source during senescence (Graham and Eastmond, 2002).
Humic acids
Humic acids are another compound that contain antioxidant properties that promote the scavenging of free radicals. The added benefits of humic acid are that they increase the availability of micronutrients, phosphate and potassium to the plant and enhance the chlorophyll content of turfgrass.
Humic acids also stimulates root initiation because of the auxin-like activity they contain, which is most likely because of their ability to inhibit indoleacetic acid oxidase breakdown (Haag, 2005; Haag and Serrato, 2006). GCI
Jeff Haag is golf course superintendent at Bowling Green (Ohio) State University. He can be reached at jhaag@bgnet.bgsu.edu.
References
Aro EM, Virgin I, Anderson B. 1993. Photoinhibition of photosystem II: inactivation, protein damage and turnover. Biochimica et Biophysica Acta 1143, 113-134.
Asada K. 1999. The water-water cycle in chloroplasts: scavenging of active oxygens and dissipation of excess photons. Annual Review of Plant Physiology and Plant Molecular Biology 50, 601-639.
Barbato R, Frizzzo A, Frisco G, Rigoni F, Giacometti GM. 1995. Degradation of the D1 protein of photosystem II reaction centre by ultraviolet-B light requires the presence of functional manganese on the donor side. European Journal of Biochemistry 227, 723-729.
Barber, J. 1984. What limits the efficiency of photosynthesis, and can there be beneficial improvements?
Barna B, Fodor J, Pogany M, Kiraly Z. 2003. Role of reactive oxygen species and antioxidants in plant disease resistance. Society of Chemical Industry, 459-464.
Bergo E, Segall A, Giacomette G, Tarantino D, Soave C, Andreucci F, Barbato R. 2003. Role of visible light in the recovery of photosystem II structure and function from ultraviolet-B stress in higher plants. Journal of Experimental Botany 54, 1665-1673.
Bilski, P., Li, M.Y., Ehrneshaft, M., Daub, M.E. and Chignell, C.F. 2000. Vitamin B6(pyridoxine) and its derivatives are efficient singlet oxygen quenchers and potential fungal antioxidants. Photochem. Photobiol. 71, 129-134.
Blokhina O, Virolainen E, Fagerstedt K. 2002. Antioxidants, oxidative damage , and oxygen deprivation stress: a review. Annals of Botany 91, 179-194.
Buchanan-Wollaston V, Earl S, Harrison E, Mathas E, Saeid N, Page T, Pink D. The molecular analysis of leaf senescence- a genomic approach. 2003. Plant Biotechnology Journal 1, 3.
Burton GW, and Ingold KU. 1984. B-carotene: an unusual type of lipid antioxidant. Science 224, 569-573.
Chen, H., and Xiong, L. 2005. Pyridoxine is required for post-embryonic root development and tolerance to osmotic and oxidative stresses. Plant Journal, 44, 396-408.
Chen Z, and Gallie DR. 2004. The ascorbic acid redox state controls guard cell signaling and stomatal movement. The Plant Cell 16, 1143-1162.
Corpas FJ, Barroso JB, del Rio L. 2001. Peroxisomes as a source of reactive oxygen species and nitric oxide signal molecules in plants. Trends in Plant Science 6, 145-150.
Couee I, Sulmon C, Gouesbet G, Amrania A. 2006 Involvement of soluble sugars in reactive species balance and responses to oxidative stress in plants. Journal of Experimental Botany 57, 449-459.
DellaPenna D, and Pogson, B. 2006. Vitamin synthesis in plants: tocopherols and carotenoids. Annual Review of Plant Biology 57, 711-738.
Delong JM, and Steffen KL. Photosynthetic function, lipid peroxidation, and a-tocopherol content in spinach leaves during exposure to UV-B radiation. Canadian Journal of Plant Science 77, 453-459.
Desikan R, A-H-Mackerness S, Hancock JT, Neill SJ. 2001. Regulation of the Arabidopsis transcriptome by oxidative stress. Plant Physiology 127, 159-172.
De Weerd, F.L., van Stokkum, I.H.M., van Amerongen, H., Dekker, J.P., and van Grondelle, R. 2002. Pathways for Engergy Transfer in the Core Light-Harvesting Complexes CP43 and CP47 of Photosystem II. Biophysical Journal 82, 1586-1597.
Drewke, C., Klein, M., Clade, D., Arenz, A., Muller, R., and Leistner, E. 1996. 4-O-phosphoryl-L-threonine, a substrate of the pdxC(serC) gene product involved in vitamin B6 biosynthesis. FEBS Letters, 390, 179-182.
Dring, M.J., Wagner, A., Boeskov, J. and Luning, K. 1996. Sensitivity of intertidal and subtital red algae to UVA and UVB radiation, as monitored by chlorophyll fluorescence measurements: influence of collection depth and season and length of irradiaton. Eur. J. Phycol. 31, 293-302.
Droge, W. 2002. Free radicals in the physiological control of cell function. Physiology Review 82, 47-95.
Ehrenshaft, M., Chung, K.R., Jenns, A.E. and Daub, M.E. 1999. Functional characterization of SOR1, a gene required for resistance to photosensitizing toxins in the fungus Cerospora nicotianae. Current Genetics, 34, 478-485.
Estevez M.S., Malanga G., and Puntarulo S. 2001. UV-B effects on antarctic Chlorella sp. Cells. Journal Photochem. Photobiol. 62, 19-25.
Flohe R, and Traber M. 1999. Vitamin E: function and metabolism. The FASEB Journal 13, 1145-1155.
Foyer CH, Trebst A, Noctor G. 2005. Protective and signaling functions of ascorbate, glutathione and tocopherol in chloroplasts. In: Demmig- Adams B, Adams, WW, eds. Advances in photosynthesis and respiration, Vol. 19. Photoprotection, photoinhibition, gene regulation, and environment.
Friso G, Spetea C, Giacometti GM, Vass I, Barbato R. 1994. Degradaton of the photosystem II reaction centre D1-protein induced by UVB radiation in isolated thylakoids. Identification and characterization of C-and N-terminal breakdown products. Biochemica et Biophysica Acta 1184, 78-84.
Friso G, Giacomelli L, Ytterberg A, Peltier J, Rudella A, Sun Q, Klaas J. In-depth analysis of the thylakoid membrane proteome of Arabidopsis thaliana chlorplasts: new proteins, new functions, and a plastid proteome database. 2004. The Plant Cell 16, 478-499.
Fryer MJ. 1992. The antioxidant effects of thylakoid vitamin E(a-tocopherol). Plant Cell Environment 15, 381-392.
Gotz, T, Windhovel U, Boger P, and Sandmann G. 1999. Protecton of photosynthesis against ultraviolet-B radiation by carotenoids in transformants of the cyanobacterium Synechococcus PCC7942. Plant Physiology 120, 599-604.
Graham I, Eastmond P. 2002 Pathways of straight and branched chain fatty acid catabolism in higher plants. Prog. Lipid Res. 41, 156-181.
Grimes HD, Perkins KK, Boss WF. 1983. Ozone degrades into hydroxyl radical under physiological conditions. Plant Physiology 72, 1016-1020.
Haag J. 2005. Preventing Summer Stress. Sportsturf, 14-19.
Haag J, Serrato C. 2006. Preventing Turfgrass Cell Damage. Superintendent, 24-29.
Havaux M, Donfils JP, Lutz C, Nyogi KK. 2002. Photodamage of the photosynthetic apparatus and its dependence on the leaf developmental stage of the NP81 arabidopsis mutant deficient in the xanthophylls cycle enzyme violaxanthin de-epoxidase. Plant Physiology 124, 273-284.
Havaux M. Eymery F, Porfirova S, Rey P, Dormann P. 2005. Vitamin E protects against photoinhibition and photooxidative stress in Arabidopsis thaliana. The Plant Cell 17, 3451-3469.
Hideg E., Kalai T., Hideg K., and Vass I. 2000. Do oxidative stress conditions impairing photosynthesis in the light manifest as photoinhibition? Phil. Trans. R. Soc. Lond. B. Biol. Sci. 355, 1511-1516.
Hortensteiner S, Wuthrich K, Matile P, Ongania K, Krautler B. 1998. The key step in chlorophyll breakdown in higher plants. Cleavage of pheophorbide a macrocycle by a monooxygenase. Journal of Biological Chemistry 271, 15335-15339.
Jeffrey, W.H. and Mitchell, D.L. 1997. Mechanisms of UV-induced DNA damage and response in marine microorganisms. Photochem. Photobiol. 65, 252-269.
Jones LW, Kok B. 1966. Photoinhibition of chloroplast reactions. Kinetics and Action spectra. Plant Physiology 41, 1037-1043.
Kariola T, Brader G, Li J, Palva E. 2005. Chlorophyllase 1, a damage control enzyme, affects the balance between defense pathways in plants. The Plant Cell 17, 282-294.
Klessig DF, Malamy J. 1994. The salicylic acid signal in plants. Plant Molecular Biology 26, 1439-1458.
Krause G.H., Schmude C., Garden H., Koroleva O.Y., and winter K. 1999. Effects of solar ultraviolet radiation on the potential efficiency of photosystem II in leaves of tropical plants. Plant Physiology 121, 1349-1358.
Krieger-Liszkay A. 2004. Singlet oxygen production in photosynthesis. Journal of Experimental Botany 56, 337-346.
Laloi C, Przybyla D, and Apel K. 2006. A genetic approach towards elucidating the biological activity of different reactive oxygen species in Arabidopsis thaliana 57, 1719-1724.
Mathis P, and Kleo J. 1973. The triplet state of B-carotene and of analog polyenes of different length. Photochem. Photobiol. 18, 343-346.
Matto AK, Falk-Hoffman H, Marder JB, Edelman M. 1984. Regulation of protein metabolism coupling of photosynthetic electron transport to in vivo degradation of the rapidly metabolized 32-kilodalton protein of the chloroplast membranes. Proceedings of the National Academy of Sciences, USA 81, 1380-1384.
Mazza C.A., Battista D., Zima A.M., Szwarcberg-Bracchitta M., Giordano C.V., Acevedo A., Scopel A.L., and Ballare C.L. 1999. The effects of solar ultraviolet-B radiation on the growth and yield of barley are accompanied by increased DNA damage and antioxidant responses. Plant Cell and Environment 22, 61-70.
McGlaughlin JC, Smith JC. 1995. Glyoxylate cycle enzyme-synthesis during the irreversible phase of senescence in cucumber cotyledons. Journal of Plant Physiology 146, 133-138.
Mitler R. 2002. Oxidative stress, antioxidants and stress tolerance. Trends in Plant Science 7: 405-410.
Mothes K. 1960. Uber das Altern der Blatter und die Moglichkeit ihrer Wiederverjungung. Naturwissenschaften 47, 337-351.
Muela A., Garcia-Bringas J.M., Arana I.I., and Barcina I.I. 2000. The effect of simulated solar radiation on Escherichia coli: The relative roles of UV-B, UV-A, and photosynthetically active radiation. Microbial Ecol. 39, 65-71.
Niogi KK. 2000. Photoprotection revisited: genetic and molecular approaches. Annual Review of Plant Physiology and Plant Molecular Biology 50, 333-359.
Noctor G, Veljovic-Jovanovic S, Driscoll S, Novitskaya L, Foyer CH. 2002. Drought and oxidative load in the leaves of C3 plants: a predominant role for photorespiration? Annals of Botany 89, 841-850.
Noctor G, and Foyer C. 1998. Ascorbate and glutathione: keeping active oxygen under control. Annual Review of Plant Physiology and Plant Molecular Biology 49, 249-279.
Powles SB. 1984. Photoinhibition of photosynthesis induced by visible light. Annual Reviews of Plant Physiology 35, 15-44.
Prasis O, Adir N, Ohad I. 1992. Dynamics of photosystem II. Mechanism of photoinhibition and recover processes. In: Barber K, ed. Topics in photosynthesis, Vol. II. The Netherlands: Elsevier 293-348.
Pruzinska A, Tanner G, Anders I, Moser S, Muller T, Ongania K, Krautler B, Youn J, Liljegren S, Hortensteiner S. 2005. Chlorophyll breakdown in senescent arabidopsis leaves. Characterization of chlorophyll catabolites and of chlorophyll catabolic enzymes involved in the degreening reaction. Plant Physiology Review 139, 52-63.
Raines CA, Paul MJ. Products of leaf primary carbon metabolism modulate the developmental programme determining plant morphology. 2006. Journal of Experimental Botany 57.
Riefler M, Novak O, Strnad M, Schmulling T. 2006. Arabidopsis cytokin receptor mutants reveal functions in shoot growth, leaf senescence, seed size, germination, root development, and cytokinin metabolism. Plant Cell 18, 40-54.
Sattler SE, Mene-Saffrane L, Farmer EE, Krischke M, Mueller MJ, DellaPenna D. 2006. Nonenzymatic lipid peroxidation reprograms gene expression and activates defense markers in arabidopsis tocopherol-deficient mutants. Plant Cell 18, 3706-3720.
Senn TL. 1987. Seaweed and Plant Growth.
Schraudner M, Moeder W, Wiese C, Van Camp W, Inze D, Langebartels C, Sandermann H Jr. 1998. Ozone-induced oxidative burst in the ozone biomonitor plant. Plant Journal 16, 235-245.
Schuster G, Timberg R, and Ohad I. 1988. Turnover of thylakoid photosystem II proteins during photinhibition of Chlamydomonas reinhardtii. European Journal of Biochemistry 177, 403-410.
Shen B, Jensen RG, Bohnert HJ. 1997. Increased resistance to oxidative stressing transgenic plants by targeting mannitol biosynthesis to chloroplast. Plant Physiology 113, 1177-1183.
Smith RC, Prezelin BB, Baker KS. 1995. Ozone depletion: ultraviolet radiation and phytoplankton biology in Antarctic waters. Science 255, 952-959.
Yoshida S, Tamaoki M, Shikano T, Nakajima N, Ogawa D, Ioki M, Aono M, Kubo A, Kamada H, Inoue Y, Saji H. 2006. Cytosolic Dehydroascorbate Reductase is Important for Ozone Tolerance in Arabidopsis thaliana. Plant and Cell Physiology 47, 304-308.
Tambussi EA, Bartoli CG, Beltrano J. Guiamet JJ, Araus JL. 2000. Oxidative damage to thylakoid proteins in water-stressed leaves of wheat(Triticum aestivum). Physiologia Plantarum 108, 398-404.
Teramura, A.H. and Ziska, L.H. 1996. Ultraviolet-B radiation and photosynthesis. In Photosynthesis and the Environment. Edited by Baker, N.R. pp. 435-450. Kluwer Academic Publishers, Dordrecht.
Trebst A, Depka B, Hollander-Czytko H. 2003. Function of B-Carotene and tocopherol in photosystem II. Zeitschrift fur NaturForschung 58c, 609-620.
Turcsanyi, E. and Vass, I. 2000. Inhibition of photosynthetic electron transport by UV-A radiation targets the photosystem II complex. Photochem. Photobiol. 72, 513-520.
Vega M.P., and Pizzaro R.A. 2000. Oxidative stress and defence mechanisms of the freshwater cladoceran Daphnia longispina exposed to UV radiation. J. Photochem. Photobiol. 54, 121-125.
Venkatrayappa T, Fletcher RA, Thompson JE. 1984. Retardation and reversal of senescence in bean leaves by benzyladenine and decapitation. Plant Cell Physiology 25, 407-418.
Von Wettstein D, Gough S, Kannangara CG. 1995. Chlorophyll Biosynthesis. The Plant Cell 7, 1039-1057.
Zheleva D, Sharma J, Panico M, Morris H, and Barber J. 1998. Isolation and Characterization of Monomeric and Dimeric CP47-Reaction Center Photosystem II Complexes. Journal of Biological Chemistry 273, 16122-16127.
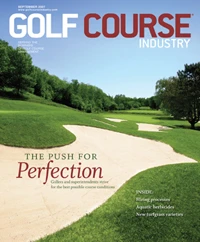
Explore the September 2007 Issue
Check out more from this issue and find your next story to read.
Latest from Golf Course Industry
- Making the grade — at or near grade
- PBI-Gordon receives local business honor
- Florida's Windsor takes environmental step
- GCSAA names Grassroots Ambassador Leadership Award winners
- Turf & Soil Diagnostics promotes Duane Otto to president
- Reel Turf Techs: Ben Herberger
- Brian Costello elected ASGCA president
- The Aquatrols Company story